Molecular machines are nano-scale assemblers that construct themselves and their surroundings into ever more complex structures. Sometimes dubbed “nanotech” in the media, these devices are promising — but also widely misunderstood. Here’s what separates the science fact from science fiction.
The concepts that underpin this form of nanotechnology have certainly had long enough to percolate through modern science. Richard Feynman first speculated about the idea of “synthesis via direct manipulation of atoms” during a talk called There’s Plenty of Room at the Bottom. Looking back, that sparked much of the subsequent thinking about treating atoms and molecules more and more like simple building blocks.
Perhaps most famously, K. Eric Drexler considered the idea of taking the bottom-up manufacturing approach to its atomic extreme in his 1986 book Engines of Creation: The Coming Era of Nanotechnology. There, he posited the idea of a nan-oscale “assembler” that could scuttle around, building copies of itself or other molecular sized objects with atomic control; one which might in turn be able to create larger and more complex structures. A kind of microscopic production line, building products from the most basic ingredients of all. Coming when it did, in the mid-eighties, it felt very much like science fiction.
Drowning in grey goo
So much so, in fact, that even Drexler acknowledged that it was prudent to tread carefully in a nano-scale building site. “Imagine such a replicator floating in a bottle of chemicals, making copies of itself,” he explains in Engines of Creation. “The first replicator assembles a copy in one thousand seconds, the two replicators then build two more in the next thousand seconds, the four build another four, and the eight build another eight. At the end of ten hours, there are not thirty-six new replicators, but over 68 billion. In less than a day, they would weigh a ton; in less than two days, they would outweigh the Earth; in another four hours, they would exceed the mass of the Sun and all the planets combined — if the bottle of chemicals hadn’t run dry long before.”
That ruthless efficiency could, Drexler argued, make some nano-robots “superior” to naturally occurring organic beings, at least in an evolutionary sense — though, crucially, not necessarily as valuable. Indeed, he suggested that omnivorous bacteria could out-compete real bacteria, reducing the biosphere to dust — or ‘grey goo’ — in a matter of days. That hypothetical end-of-the-world scenario, where nanobots turn our world and us into an amorphous sludge, was as tempting to sceptics as the promise of nanotechnology was to scientists. Still, almost thirty years on we’re still here and, while some of us may be a little more ashen of face, we’re yet to be submerged in the biological by-product of engineered molecular machines.
Building blocks
Truth is that scientists have been very busy indeed over those past thirty years, creating a host of molecular-sized structures that can manipulate and assemble themselves, move, and even work together. It’s not always easy, of course — building at the molecular levels requires atomic accuracy — but mercifully chemistry and physics has advanced to a point where it’s increasingly possible. And there’s a rich pool of molecular machines, some inspired by nature, others by mechanical engineering principles, to show for it.
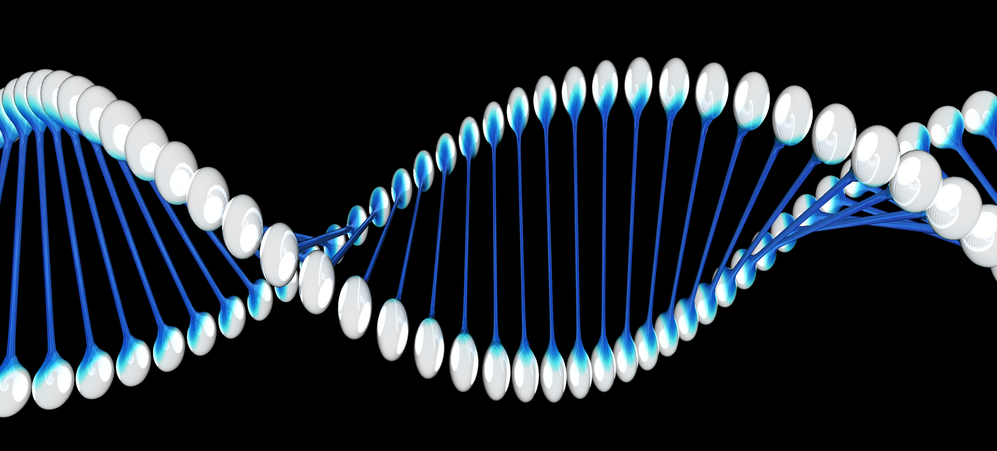
The majority of successes have been built from DNA molecules. Here, DNA isn’t being used to carry genetic information; rather, it’s a structural material in its own right. Its four bases — adenine, cytosine, guanine and thymine — bind more or less strongly to one another depending on how they’re paired up along the length of a DNA double helix, allowing scientists to tweak the way in which they join together. “We can direct the associations of molecules through Watson-Crick base pairing. Intermolecular interactions using sticky ends have a well-defined geometry,” explains Professor Ned Seeman, a nanotechnologist in the Department of Chemistry at New York University, who’s widely regarded as inventing the field of DNA nanotechnology. “DNA is like Lego.”
The fundamental building blocks of life already have the features required to fold, join, build and grow — so they’re perfectly suited to building things at the nano-scale. By creating strands of DNA with carefully controlled base sequences, the binding can be specifically tailored so that customised strands can be combined to bind with each other and construct exotic structures. Geometries are first modelled on computers to work out what molecules are required, then the appropriate can be DNA synthesised in order that they can be put together — just like a Lego kit.
No assembly required
But unlike Lego, when combined in solution the DNA can form structures without intervention. The interactions between strands are controlled by their base sequences: there’s a preference for certain sites to bind and others not, resulting in a glorious self-assembly process. If Drexler’s suggestions of assemblers felt like science fiction in the mid-eighties, then the fact that molecules can be designed to build themselves into new and complex structures surely renders it fact.
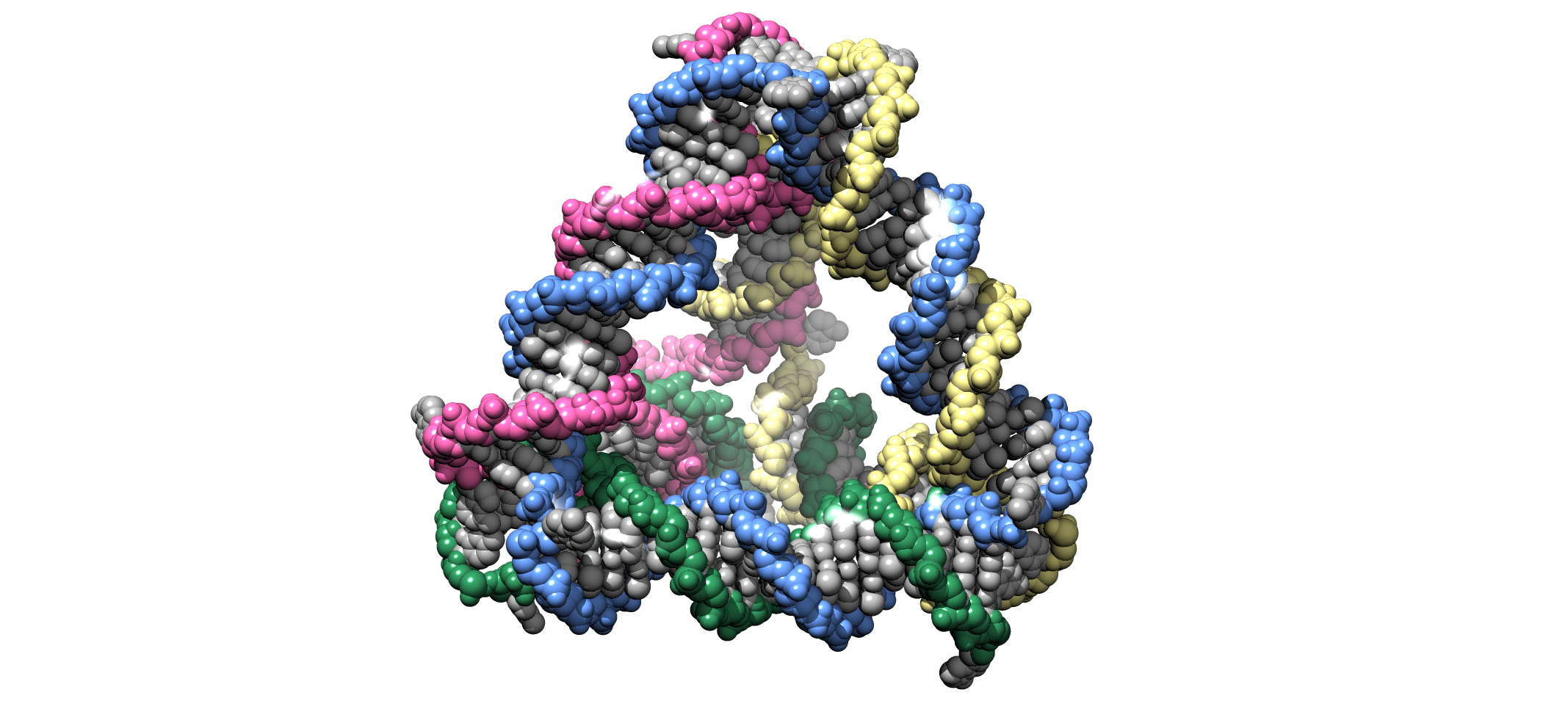
Indeed, Seeman’s lab has a rich history of creating self-assembling complex objects such as crystals out of a simple puddle of DNA molecules. His labs have created 2D and 3D crystals, as well as a wide variety of geometric shapes using the techniques. There are plenty of other researchers working in the area, too. Professor Andrew Turberfield at the University of Oxford, for instance, uses DNA molecules to create individual tetraheada, such as the one pictured above. Mixing four different types of DNA, each designed to join together in pre-defined ways, his researchers can create tetrahedra with 7 nanometre edges. They can be used to lock proteins inside the structure, to be deployed to an area where medical treatment is required — a kind of assembling and self-deploying container system at the molecular level.
Make your move, molecule
But the slew of nano-engineered molecules don’t just assemble themselves — they move, too. A number of research groups have created molecules that can walk, much like humans or animals. Synthesised from DNA, they’re supposed to walk directionally along a track, though until recently it’s been difficult to accurately gauge whether the walkers had ‘jumped’ or ‘floated’ to a new location — because the steps they take, at around a nanometre in length, are hard to detect using traditional techniques. Fortunately, researchers from Oxford University’s Department of Chemistry have now laced the walker’s DNA with arsenic, and are able to track it as it leeches through a porous track — proving once and for all that the walkers do indeed do as they’re supposed to.
Elsewhere, mechanical engineering is a large influence on construction — hence the popular moniker molecular machines. Motors, for instance — one of the life-size objects we largely take for granted — have assumed molecular form. The first molecular motor was made in 2021; the fastest yet produced just last year. The smallest simply spins about a sulphur atom while sat atop a clean copper surface — reaching speed of up to 7200 revolutions per minute. The fastest, made of a rather more bulky three molecular components, can reach speeds of 18,000 revolutions per minute — roughly the same speed as a jet engine.
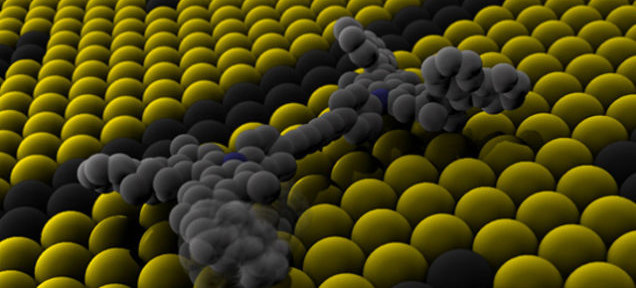
Perhaps the most complete example of a molecular machine so far, though, is the nanocar, developed by a team of Netherlands-based researchers. Made up of a long central body with pivoted paddles at each of its four corners, a pulse of electrons can be used to swing the paddles around in circles, a whole quarter turn at time. That quarter turn puts the molecule in to an unnatural arrangement, so the bonds continue to move another quarter turn to reach a state of equilibrium. To keep the car moving requires a pulse of electrons every half turn. It’s perhaps not setting any distance records — it takes 10 pulses of electrons to move the vehicle 6 nanometres — but it is a molecular car. Give it a break.
Alternative energy
All this raises an easily overlooked problem though: how do we power these molecular machines? “The major challenge so far in the field is still the propulsion of synthetic nanomotors,” explains Dr Wei Gao, from the Department of Electrical Engineering & Computer Sciences at the University of California, Berkeley. “New nanomotors which can travel inside living animals, especially the bloodstream, efficiently are still strongly desired.”
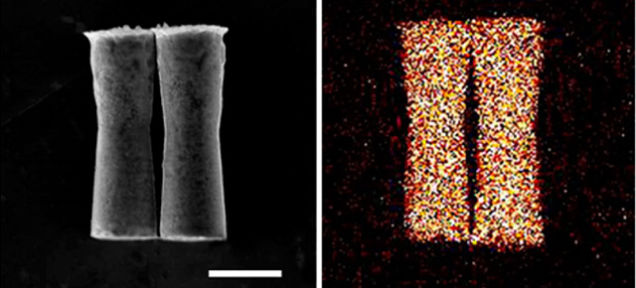
The motors and cars described above typically use a stream of electrons for power — usually delivered by a tunnelling electron microscope to deliver them with precision. But that’s not a feasible sources of power for any application outside the lab, and certainly not inside the human body, so researchers like Gao have been developing alternatives. He’s recently created a new kind of 20 micrometre-long polymer tube — pictured above — that’s coated with zinc. When it finds itself in an acidic environment, such as the stomach, the zinc reacts to form hydrogen bubbles, which in turn propel it forward with enough velocity to lodge itself into tissue — delivering a payload of drugs with it.
That’s a fairly crude and undirected form of propulsion, admittedly — but it does prove that molecular structures could exploit their surroundings to create the energy required to power them. “Synthetic nanomotors requires external chemical fuels which can greatly hinder their practical use,” explains Gao. “Zinc-based nanomotors are the first example of nanomotors which use the their natural surrounding as the fuel source. In addition, we developed biodegradable magnesium-based nanomotors, which can use water as the fuel in presence of chlorine ions. We could imagine that the future nanomotors can be self-propelled in diverse bio-fluids such as human blood.”
Put it all together
There is, clearly, no shortage of technology at the nano-scale. We’ve self-assembling structures, folding shapes that can collect and release loads, walking contraptions, molecular motors, vehicles that make use of them, and, perhaps, even the potential to fuel them. You can also add to that list a smattering of smart extras, too — like single-molecule LEDs and simple DNA-based computers — that could, one day, make them more than just simple mechanical systems.
So is there any hope of Drexler’s nanoscale production line? “We’ve already shown that,” explains Seeman, as if it were never really in doubt. A few years ago, Seeman’s lab showed off what was the first molecular production line. Four DNA nanorobots walked along a specially-prepared surface, picking up gold particles and interacting with each as they moved to build themselves into one of eight different end products. Admittedly the finally result was one of eight different tangles of DNA and gold particles, but as a proof of concept it was irresistible.
Progress hasn’t halted since. Other assembly lines have been created which, instead of building a tangle of DNA, actually piece together smaller molecules to form larger, more complex structures that can then be used for an entirely new applications. Those molecular motors have been put to work, too, using their rotational abilities to control chiral catalysts — molecules that themselves speed up other reactions, depending on the orientations of the atoms within the more complex structure. Molecular machines are being used in labs the world over to tweak and assemble other molecules, creating larger assemblies that can be used for another purpose.
Fiction to fact
In other words: we’re getting there. But instead of the primordial stew of nanobots producing grey goo, the reality of the molecular building site is a more nuanced, structured and modest one. Perhaps its no surprise that Drexler himself has tweaked his own hypothesis about the future on nanotechnology. In his 2013 book Radical Abundance, he writes about “microblocks”. These, he suggests, are a kind of Russian Doll assembly process, where a cascade of thousands of tiny robotic cells each builds components at the molecular level, feeding those components to increasingly larger robots until a full-size product is created. You can imagine it as a series of ever larger 3D printers, the first working at the molecular level, each creating components to pass up to the chain, to create larger and larger structures.
Of course, with that kind of increasingly complexity comes greater challenge — but given the distance we’ve come, such obstacles shouldn’t be seen as too daunting. “Life itself is not possible without the multitude of molecular machines inside our bodies that work in turbulent environments and with very little power but accomplish very complex tasks from virus transport to muscle flexing,” explains Professor Charles Sykes from the Department of Chemistry at Tufts University. “Many wonderful things are possible as nature has already proven.” Indeed, as academics see it, there’s only a single real barrier. “The key constraint is the usual one,” muses Seeman. “Money.”
Really, then, the revelatory ambitions of the eighties assemblers have simply mellowed. Rather than scuttling around making — or destroying — at will, the molecular machines of the near future will be ordered, constrained and working for us rather than in competition. Rather less alarmist, and rather more useful. And that, it seems, is what happens when science fiction becomes more like science fact.
Pictures: microvector/Shutterstock, Turberfield, Wei Gao, University of Groningen, Guru 3/Sutterstock